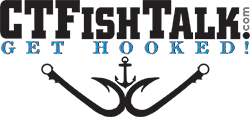 |
|
View previous topic :: View next topic |
Author |
Message |
BlueChip
Joined: 29 Jun 2011 Posts: 177 Location: New Haven/Madison/Essex
|
Posted: Thu Oct 02, 2014 10:23 am Post subject: Black Mayonnaise Habitat & Marine Diseases 1972-present |
|
|
Black Mayonnaise Habitat and Marine Diseases 1972 to Present
IMEP #28
Habitat Information For Fishers and Fishery Area Managers
Understanding Science Through History
(IMEP Habitat History Newsletters can be found indexed by date on
The Blue Crab.Info™ website: Fishing, eeling and oystering thread
And Connecticut Fish Talk.com Salt Water Reports)
The Role of Dredging, Flushing and Increased Tidal Exchange for Habitat Restoration
Are “Dead Zones” of Poorly Flushed Coves and Bays Natural or Unnatural
Revised from Not All Dredging is Bad 2009
A Habitat History for Nitrogen Containing Sapropel 2011
Eastern Long Island Sound Supplemental Environment Impact Statement –
Dredged Material Disposal Site Comments
Comments from Tim Visel
Submitted to Alicia Grimaldi – December 20, 2012
Ocean and Coastal Protection Office Environmental Protection Agency
Region 1, Boston, Mass 02109-3912
Revised for Sapropel Capstone Research Project Catalog
September 2014
The Sound School, New Haven CT
Attention Blue Crabbers – A Caution Regarding Black Mayonnaise Habitats
Note – September 2014 – Tim Visel
Since this paper was submitted to Region 1 EPA’s office regarding “black mayonnaise” or Sapropel bottoms and dredging disposal several new reports have high highlighted new or dangerous bacteria strains in or near them. People are becoming sick when exposed to several Vibrio strains of bacteria which have increased following heavy rains and warm temperatures. Could the increase in Black Mayonnaise bottoms be connected to diseases of fish, shellfish and even us? I believe this is so. Commercial and recreational shellfishers raised the first alarm bells decades ago.
I can recall talking to Jake Dykstra, a longtime trawler captain and commercial fishing vessel owner in Rhode Island about reports of lobster shell disease caught in or near the New York Bridge dump site (1977-7 . He described large areas of the bottom then covered in a “black sludge” with lobsters that had shell disease, sometimes called shell pox, back then. Articles in National Fisherman™ highlighted the conditions of the dumpsite sludge (during the 1970s this relatively shallow dumpsite was receiving some 50,000 tons of animal fats and grease each year). I had never seen any evidence of lobster shell disease while lobstering in Connecticut from 1967 (Shell disease appeared in western Long Island Sound lobsters in 1990) so reports of this was perplexing at the time. In the early 1980s black mayonnaise had already come into shallow areas – low energy reduced (tidal exchange or flushing) and much warmer such as Tom’s Creek in Madison. With the appearance of black mayonnaise came several unwanted quests. One of the first signals was the appearance of a parasitic trematode worm (Schistosomasis) on clam flats in Westbrook, CT in 1978. By August 30th of that year the Shoreline Clinic in Westbrook had treated “more than ten cases of swimmers itch already, this summer according to clinic Director Dr. Gordon Van Ness.” Director Van Ness noted that those patients treated “had all been clamming along mud flats in Westbrook” (The Day, August 30th 1978 pg 2).
Besides smothering the shellfish in the creek, this new problem with shellfishing appeared today called channel itch or swimmer’s itch (clammer’s itch), which is a nice way of saying blood parasite, now invaded the shore areas of Clinton, Madison and Guilford and people’s skin in the early 1980s. This parasite is vectored by the way of the mud snail (who were also feeding along the edges of now deepening mud / mayonnaise deposits) of the Hammonasett River. Within a few years of black mayonnaise MSX appeared in central Connecticut and wiped out most of the oysters in the same area, starting with shallow areas such as Tom’s Creek and those areas that had black mayonnaise first. At the same time winter flounder fishers started bringing me (at UConn in the 1980s) winter flounder with necrotic fins and rotting flesh wounds; out of all the years of winter flounder hand-hauled trawling off of Madison on sandy bottoms I had never seen large flounder with gaping, oozing wounds. The incidence of fin rot increased in shallow areas, now covered with “black mayonnaise.” As the summer temperatures increased into the 1980s the incidences of winter flounder fish rot increased. The winter flounder caught on muddy bottoms had the highest incidents of fin rot – such as the Morris Cove section of New Haven Harbor.
Year after year enormous quantities of leaves now washed into Connecticut’s estuaries and New England in general. Black mayonnaise soon covered bottoms on Cape Cod and many coves, which was followed by habitat failure characteristics for winter flounders and bay scallops into eastern New York as well. Lobsters, hard shell clams, oysters and winter flounder all suffered disease outbreaks.
The past few years have now seen some hideous flesh eating diseases, mostly associated with fin and shellfishers (including blue crabbers), again in the shallows and those areas most impacted by heat and organic loadings after cuts or punctures. This is far worse then some of the lobster industries’ “bait wounds” also called “fish poisoning” (blood poisoning) of the 1960s (I speak from personal experience here. Dr. Norman Rindge of Clinton saved my right index finger in 1969) and the regions most impacted are very warm shallow areas often containing soft, black organic deposits. Do I think that these disease events are related to the presence of black mayonnaise habitats? Yes I do, absolutely.
This paper was first written regarding dredging site contaminant and dredging as habitat improvement process not as pathology of disease vectors. However, it mentions some of the processes and habitat characteristics of Black Mayonnaise habitats. It also contains some of the basic chemical processes associated with black mayonnaise deposits (Sapropel to the scientific community). When oxygen is introduced the bacterial strains in these deposits die, dredging or storms can also end them. Sulfur reducing organic deposits are known to contain some parasites and very dangerous bacterial strains. As far back as 1974 tests showed several (sludge deposits) had bacterial strains resistant to all five known antibiotics (O’Connor 1975 Contaminant Effects on Biota of the New York Bight, p.61). They are now linked to shellfish and finfish disease and under certain conditions can even become deadly to us.
Sharp oyster shells have cut some blue crabbers here Connecticut and I have seen two wounds recently that I have urged people to have checked out immediately.
Increase in the blood fluke – Snail Fever has also occurred in the Clinton Connecticut area last month.
Everyone who works or fishes in the shallows in the presence of black mayonnaise should be very careful of “muddy cuts” and the presence of blood flukes (snail fever).
Tim Visel – I respond to all emails – tim.visel@new-haven.k12.ct.us
Revised to Capstone Project Title – Did Black Mayonnaise Habitats Bring Disease?
Introduction
Some basic questions regarding the health of Long Island Sound were asked by coastal residents especially by those who fish, shellfish lobster and crab in the 1980s.
Is habitat quality subject to climate and energy impacts in Long Island Sound? And, is flushing related to the strength and severity of anoxic conditions in Western Long Island Sound? A quick review of the 1974 to 2004 period will show massive habitat shifts as reported by coastal fishers. In almost every instance fisheries changes occurred after observed habitat changes. The New England inshore fisheries, especially those in coves and bays, user group (fishers) comments included concerns about these habitat changes. Nearly all of them spoke about the “bottom” previously firm or hard bottoms have now become softer, (composting) and often muck filled. As these changes occurred, the fishery associated with them also changed, they declined. Chief among them would be winter flounder, bay scallops and the hard clam. At the same time, the boating community also noticed changes often as lessening depths and the need to conduct navigational dredging projects to maintain channels. Navigation soon became difficult then impossible in many small tidal rivers. A black greasy accumulation covered many shellfish beds and later winter flounder developed fin rot, MSX outbreaks devastated oyster populations followed by lobster shell disease and then fresh eating bacteria. The previously hard bottom habitats were transitioning into softer ones. Some of the first mention of lobster shell disease chitin consuming bacteria came from Rhode Island offshore lobster fishers near the New York 10 miles Bight dumpsite in 1977.
These user group accounts are consistent from the baymen of eastern Long Island, Rhode Island’s South Shore (salt ponds), Connecticut and Cape Cod, Massachusetts. Frequent observations in the late 1970s to 1980s mentions white films or fungus growths on bay bottoms that in years past, were firm and shelly, especially those on eastern Long Island, including Peconic Bay New York. Here small boat fishermen who once hand hauled otter trawls for winter flounder and those who bay scalloped were among the first to notice these habitat shifts. In areas that were once clear and firm, now contained deepening organic deposits turned black and foul bottoms that often smelled especially during summers of rotten eggs. Over time, these vegetation deposits included sea grasses decayed leaves and seaweeds, and were more than inches deep in the more sluggish coves – and it soon would be measured in feet (Lewis Bay Hyannis Massachusetts 1983).
As depths decreased flushing capacity lessened and in time shellfish habitats would soon become buried in a marine compost, Sapropel. Storm energy declines mirrored the increase of Sapropel killing marine life and altering subtidal habitats after 1972. That also it seems brought marine diseases by 1978.
Diseases associated with warm organic deposits - sludge
The presence of increased bacteria decreased biological activity and increasing diseases have been known for quite time regarding this habitat type. In 1975 Joe O’Connor of NOAA found in some of the first New York Bight (sludge) studies antibiotic resistant bacteria. He reported that the New York Bight area had high winter flounder fin rot incidences but nearby sandy bottoms almost nonexistent and experienced “none of the crustacean (crabs and lobsters) held on clean sands developed any pathology” (disease). In Boston Harbor William Tobin (1998) found that accumulating “sludge” was advancing from the mouth of rivers (1968) not adjacent to sewer out falls (most likely from Tannin – Sapropel formation mentioned in this report). The same report (Sustainable Management for Dams and Waters 1980) found that winter flounder fin rot incidences had reached one of the highest levels ever recorded, 44% in some parts of Boston Harbor. The source of this sludge could be human waste or organic wastes and it was not just on the east coast; this habitat battle on the bottom was noticed out west as well Dr. Dosey monitoring a sewer outfall in Santa Monica Bay had in 1984 reported that diversity dropped nearly 50% next to an outfall containing sludge from sewage. He also termed it black mayonnaise. Diversity (the number of different organisms found in given area or habitat type) was much less on sludge (black mayonnaise) than other parts of Santa Monica Bay (source, Bill Sharpstein’s “Dirty Water”). Fishers in Chesapeake Bay after Agnes in 1972 also noticed an increase in “black bottoms,” no doubt also deposited during heavy rainfall source, US Army Corps of Engineers, The Effect of Hurricane Agnes, June 1973. Most likely proceeded by a release of tannins (brown waters) followed by a sulfide block (not reported in 1973 report).
Core samples of the sediment profile was taken to measure deposition 1972-73 but results were not included in the January 1973 report – monthly core samples were to be taken to answer many questions about sediment physical and chemical characteristics. One of the questions raised in the 1973 Army Corps report was will there be subsequent undesirable algal blooms after. As a result of Agnes rains (Wikipedia source material) which recorded record flow rates for the Susaquehanna River at the Conowingo Dam which for a time seemed unable to cope with normal flood controls – requiring charges to be positioned to blow our a section of the dam to prevent catastrophic failure if needed.
Between June 21st and 23rd 15” inches of rain fell central/eastern Pennsylvania creating record floods and large loss of life. Low salinities was very damaging to the Bays oyster industry and both soft shell clam fisheries and blue crabbing declined to 1976. It is curious that many studies of the Chesapeake Bay after Agnes – no specific references can be found to organic matter in any form. After 1972 the coast of New England was to experience red tides. H.H. Selger et al 1975, Dinoflagellate Accumulation in Chesapeake Bay studied gymnodium breve (red tide) and proposed the possibility that non toxic Dlnoflagellate accumulation can serve as nutrient sources for fish pathogenic bacteria and thus “precipitate a self regenerative massive fish kill pg 201. Much of the bacteria then was associated with “mud bottoms.” One aspect however was the appearance of mahogany tides (known today as chocolate waters) followed by red phylo plankton blooms. A major soft shell clam die off occurred July 11 and some commercial crabbers reported dead blue crabs in pots pg 114. Crab kills then were attributed to a HAB harmful algal gymnodium splenders a substantial blue crab kill in Chesapeake Bay in 1971 was attributed to low oxygen high temperatures and bacterium Vibrio “parahaemolytius.”
Dan Fisher of the University of Maryland Biological Laboratory found that these (sludge) areas did not contain much organic life “except a species of bacteria that didn’t need oxygen.” (Saving the Bay, Ann E. Byrnes, 2001).
Coastal Storms Deposit Huge amounts of Terrestrial Debris
Dredging coastal salt ponds, maintenance channel dredging and clearing mooring basins is not that different than that of tidal inlet flushing from storm events. A natural energy process that “restores” previous depths, providing safer access for boating and navigation interests but it helps restore habitat conditions for fish and shellfish species. Dredging the build up of marine composts (which is a often toxic sulfide rich gelatinous material), can improve habitat quality. We need to be able to move such deposits (organic rich matter) in oxygen deficit areas into those that are oxygen sufficient. Dredging may be one of the few tools we have in the climate change tool box to increase tidal circulation and enhance dissolved oxygen water exchange. Dredging to restore tidal flushing/tidal exchange will also enhance shellfish and finfish habitats in two important ways enhance the capacity of higher pH ocean water to offset flow pH microbial deposition and reduction processes (The Sulfur Cycle). Cycle Mackenzie of NOAA once described a small dredging project to improve bay scallops habitat – it worked.
Not All Dredging is Bad
Dredging can also eliminate nitrogen “banks” accumulating nitrogen compounds that bind to these organic low pH mucks. During hot periods and low energy nitrogen is naturally stored in these mucks which can take centuries to clear. Recent studies show that in high heat Sapropel can shed enormous quantities of ammonia. Dredging may reduce the nitrogen residence time by decades even perhaps centuries. While nitrogen pollution has been at the forefront of environmental policy, it has not been correctly indexed to temperature and energy. Therefore dredging can mechanically remove nitrogen rich deposits, restore flushing and provide navigable waters. To do so, however, will require deposal sites for this sulfur rich material (Acidic Sulfate Soil) and in oxygen sufficient waters where oxygen reducing bacteria can reduce it and it can reenter the marine food chain (fish food). The key to reducing sulfur toxicity is to restore oxygen dependent reduction processes. Dredge material disposal sites will have a key role in this process.
Many pollution studies have previously examined the nitrogen issue, but very few mentioned the time it takes for nitrogen to clear naturally; it may prove cheaper and certainly quicker to dredge the excess. To allow natural processes to clear excess nitrogen which naturally accumulates during periods of warmth (sulfur reduction) and is utilized during cold (oxygen reduction) may take decades or even centuries. Quick recoveries of living marine resources therefore should not be equated to aqueous nitrogen abatement.
In a 1971 book by H.B.N. Tynes Professor of Biology University of Waterloo Ontario, Canada, he warns researchers about promising quick recoveries following eutrophic conditions. In lake studies he describes this nitrogen banking processes and the time it takes to clear it. Most lakes and ponds are periodically dredged to quicken this habitat recovery process. In a recent NOAA study by Clyde Mackenzie who looked at tidal areas for hard shell clam production (Mercenaria mercenaria) found that production was less when ocean tidal exchange restricts (smaller inlet width) but production (clam landings) soon increased (sometimes dramatically) when tidal exchange (flushing) was increased due to inlet widening (after storms) or by dredging (see appendix).
Dredging may directly remove low pH acidic deposits (especially from acidic oak and maple leaves) in areas where sulfur reduction (sulfate reducing bacteria – sulfur reducing bacteria) is building huge nitrogen reserves. In high heat these composts reduce producing ammonium, a plant nutrient that favors the growth of algae “blooms”. Some of them are harmful to shellfish species (HAB). In poorly flushed coves or bays that have restricted circulation low oxygen levels and a heat induced low pH combine to lock up nitrogen compounds in enriched organic matter preventing it from entering estuarine food webs. After storms oxygen is reintroduced into this material creating release of sulfide waters (called black waters) and general acidic conditions from sulfuric acid generation. (These can reverse habitat conditions when oxygen is now not limiting).
The boating community has often reported such changes damages metal struts apart from electrolyses. Shallow water observations of depths had decreased and bottoms now deep in muck often smelled bad (hydrogen sulfide) similar to comments from fishers. A previously minor nitrogen input (leaves) during cold and energy periods can be devastating during high heat and less energy. Hot oxygen reduced leaf “composts” in the marine environment is now a huge source of ammonium, and as damaging or more so than human nitrogen discharges. The building up of sulfide rich acidic organic deposits has resulted in wide scale habitat degradation and could take centuries to clear localized ecosystems. Dredging could help speed this process
In times of high heat dissolved oxygen in sea water drops and areas that are poorly flushed may suffer seasonal hypoxia. For many shallow water bodies this appears to be a natural cyclic ecosystem event. Long Island Sound most likely experienced hypoxic episodes many times before leaving the cold and turbulent 1950s. Termed the North Atlantic Oscillation (1950 to 1965) this period is remembered by colder than average winters and at times unbelievable levels of storm activity. Colder waters allowed dissolved oxygen levels to increase – oxygen reduction quickly utilized organic debris as nitrogen compounds and quickly washed it from bay bottoms. With the cold and storms, nitrogen in Long Island Sound became limiting. In fact, research was underway at Yale University to determine the extent of the nitrogen shortfalls, it was suggested that for a time, nitrogen became limiting in Long Island Sound. The climate had much to do with this 1950s nitrogen “shortage” as organics such as today leaves woody debris and terrestrial nitrogen sources. In cold periods Nitrogen did not “bank” in partially reduced composting accumulations. Although many marine studies label them as sediments or even soils, that is a misnomer, as much as you would label leaf compost, a soil in terrestrial ecosystems.
As such terrestrial accumulations are transitory and in time sufficient oxygen and bacterial processes will breakdown leafy material into soil components. However, three feet of leaves is not a soil or similar unreduced organic matter be termed sediments in marine ecosystems. Many dredging projects therefore are merely compost removal activities. It is safe to say that even without our nitrogen inputs – shallow warm poorly flushed bodies of water undergo periodic climate induced hypoxia, and fish kills and algae blooms from high heat and low energy conditions are as old as recorded time itself.
Physical and Chemical “Erosion”
During warm and low energy periods sand dunes tend to grow – plants soon “invade” and hold the sand in a banking process, the sand dune itself. Warm water is naturally less dense and has a different erosion capacity, in fact, periodic energy during warm periods tends to move sand bars ashore and seasonal winter – summer beach profiles often show this sand bar movement back out. The same could be said for Sapropel storing nitrogen.
When a cold and energy filled period commences, tides, waves and strong storms tend to draw against this sand “bank”. We can see this withdrawal from this sand reserve as beach erosion, as fishers often report Sapropel “melting away” to black sands below.
Since our current sea level rise period is hundreds of years old, we can see from today’s nautical charts the shorelines of long ago when they ran out of banked sand. They are the near coastal depth contours. When the sand dune bank ran out, the sea claimed the property below them as it had since the last Ice Age, as a natural process. When the same happens to Sapropel it erodes from oxygen respiration reduction (Chemical Erosion). There is no short term dynamic equilibrium but a long term fluctuation since the last Ice Age dictated by temperature and energy cycles.
During warm and low energy periods, organics tend to bank in the shallow poorly flushed areas. These are the same areas that contain essential fish and shellfish habitats, the ones also user groups historically observe. This is the habitat transition (reversal) found so frequently in fisheries reports – the change for firm “hard” bottoms, often with estuarine shell, a natural pH buffering agent. This change from an alkaline to acidic marine soil has dramatic consequences for estuarine organisms, bivalve sets decrease, winter flounder habitat becomes too acidic and the red macroalgae plants give way to acid tolerant ones especially eelgrass, Zostera marina. The ability of eelgrass to trap organic matter many times as dense as bare sand has a huge role in the acidification of marine soils. Its ability to trap organic matter in high heat adds to the rapid rise of the bottom profile. Much of this influence is from terrestrial inputs as detritus dead organic matter, leaves, woody debris and dead grasses from land. Eelgrass blades trap this debris (called oatmeal by fishers) a brown loose easily disturbed “chaf” which fills shores between sandbars and forms in tidal eddies and in high heat stimulates the sulfur reduction cycle. High heat drives oxygen from these shallow waters (inverse solubility law) and different types of bacteria soon dominate; the sulfate and sulfur reducing bacteria (many strains and species). As the oxygen level drops oxygen dependent decomposers are soon overwhelmed and this organic matter is now “banked” as an accumulation of viscous jelly like material (again not a soil or sediment) but as partially reduced “marine compost” or Sapropel. Some of these new sulfate reducers are not kind to previous hosts or habitats.
Some of the direct negative consequences is the removal of all chemical available oxygen – if oxygen was “sand” on a beach it would disappear as waves carried it away. If sufficient oxygen is available oxygen reducers consume the organic matter into components more digestible although growing by oxygen organisms such as shrimp species. Remove the oxygen and the consequences of this exchange become apparent. No longer is oxygen sufficient for marine life becomes limiting and sulfur reducing bacteria increase deep below the accumulations out of the zone of oxygen influence. As Sapropel deepens the sulfur reducing bacteria increase digesting organic matter that in the process shed ammonia, complexes hydrogen sulfide (which is very toxic to marine life) and sets up the mechanism for sulfuric acid and sulfide washes often storms. The mechanical energy of storms and waves tend to rip open Sapropel exposing years (sometimes decades of sulfur compounds) into a black or grey water event. Fishers often report the smell of sulfide (sulfur) days after a storm or fish kill. One of my Oceanography teachers at Florida Institute of Technology had a quick verse –
“If you can smell it, the fish have felt it”
This is true after heavy rains disturbing previous Sapropel deposits while creating or bring the organic matter to new areas to begin the sulfide process especially in hot weather when oxygen is limiting. This is largely a natural process that we can enhance by making organic matter into an oxygen limiting environment and is the basis of the century old Saprobien system.
When Sapropel is exposed to oxygen again it quickly reverses sulfide to sulfuric acid killing many organisms from extremely low pH creating the sour or acid bottoms frequently mentioning by fishers. Sulfuric acids in marine soils have been known to reduce or eliminate shellfish sets (Belding 1912) and plagued terrestrial farmers who harvested deposits for fertilizer.
Sulfur reduction in (warm hot) estuarine can kill larval farms upon contact, including shellfish veligers and blue crab and lobster megalops. In cold water sulfide levels build up as a natural process killing fish under ice – frequently in salt ponds. These events are frequently proceeded by the smells of sulfur.
Excess or the build up of organic matter is deadly to the fish and shellfish harvests we “value.” Alewife fishers noticed the impacts of cut off salt ponds – those ponds that had entrance blocked and therefore removed from daily flushing – organic mater out and oxygen in. Blocked salt ponds would suffer some of the first black water fish kills. Coastal residents (those who valued the shellfish and finfish in these ponds) would unblock them.
This organic matter build up would also influence navigation. Some of the recent investigations include navigation as a habitat restoration tool, marinas and boatyards who remove leaf build up have reduced oxygen tension (demands) in fact often many marine dredging projects “fishing” usually improves. That is why.
Estuaries can hold this banked organic matter we can observe as decreasing depths. Decades ago people realized the impact of these accumulating leaves and would upon leaving channels drag iron rings or old metal frames to loosen and dislodge these rotting leaves on outgoing tides, removing them from oxygen depleted channels to the more oxygen sufficient open waters of Long Island Sound. Later this practice would also be termed prop washing, but it wasn’t really that different than oxygen injection into waste water treatment plants or flushing bio filters to reduce biological oxygen demand – same process just a different scale.
Oxygen depletion does influence the organic deposition accumulation rate, the lower the oxygen the faster this organic material (and nitrogen compounds) is banked. It is not unlike the process of land locked water bodies, lakes and ponds which accumulate over time this organic compost (colonial farmers would frequently harvest this compost for terrestrial soil nourishment) builds up and pond/lake depths decrease over time, removal accomplished by storms (floods) or our intervention – dredging.
With a renewed and vigorous forest canopy in Connecticut this process occurs in the coastal environment also especially in times of extended heat. It is this “marine compost” that fishers (shellfishers especially) noticed accumulate on previously hard or clear (and often deeper) bottoms. In times of heat this process starts slowly a few inches but as the material becomes acidic and sulfur rich this process quickens reaching several feet. It is then banked rich in plant nutrients (nitrogen) and phosphorus that could last hundreds of years. In fact, much of the nitrogen ammonium compounds and phosphorus spring “flush” is the result of decayed leaf materials washed down brooks and streams into the estuaries. The restored forest canopy trees can alter the nitrogen retention process tilting it toward the sulfide reducing bacteria made infamous for the “stink” of salt marshes here in CT during an extremely warm periods and few storms, during the so called Great Heat 1880-1920. It is at this time that marsh stinks were linked briefly to “bad airs” and disease vectors, but what really were smelling was strong hydrogen sulfide gas emitted during the sulfur reduction process in high heat and low oxygen. Thus the rotten egg odor at the turn of the century usually occurred in late August during the height of the summer heat. At the turn of the century many coastal Connecticut towns reported strong rotten egg smells emanating from salt marshes during this period (1880-1920).
Because it is difficult to see this process, these reports labeled the marshes as the culprit, but in actual fact it was the decomposition of organic material sealed from the atmosphere, those deposits under the water. It is also the time of the immense juvenile winter flounder fish kills of eastern New York in bays and coves high heat sulfur reducing bacteria can change the chemical and biological characteristics of this “banked” organic material, it now tends to become acidic by the release of hydrogen ions and soluble metals to be converted into insoluble metal sulfides. That is why metal levels appear to rise over time in these oxygen depleted areas.
In a 1980s mining case history and in experiments conducted by EPA, scientists confirmed the metal recycling ability of sulfate-reducing bacteria that chemically convert dissolved metals into insoluble metal sulfides. Therefore, in high heat/low energy conditions, deep accumulations of organic matter become rich in metals over time. Thus, in these high heat/organic prevalent deposits, metal levels will naturally increase. The longer sulfate reducing bacteria affinity (potential) to reducing bacteria exits, it can complex them in this oxygen deficient organic matter. This appears to be part of the natural mineral salt accumulating process. This natural metal complexing process has confounded numerous dredging projects in low salinity areas found in nearly all Connecticut’s rivers. I have found a quick chart showing the potential of sulfate-reducing bacteria to complex heavy metals – from a 2003 paper that looked at bio remediation of acidic mine waste water.
Percent Recovery of Metals from Mine Water (waste water) Using Sulfate-Reducing Bacteria
Metal Percent Recovery
Aluminum 99.8 Many organic deposits below salt marshes have high levels
Copper 99.8
Zinc 100.0 Zinc taste often appears in oysters
Cadmium 99.7
Cobalt 99.1
Iron * 97.1 As such, many mine waste waters with reduced pH will appear red (black in 02
Maganese 87.4
Nickel 47.8
*See associated oxidation of ferric hydroxide (ochre)
This chart is from an EPA study – Takak, Henry H., et all (2003) Bio-degradation 14:423-436 as found in a college textbook Environment: The Science Behind the Story (page 657).
One could expect that aside from tank studies conducted by Takak (2003), this process occurs in nature under high heat and low energy (mixing) of oxygen sufficient waters above. Field surveys of deep deposits of partially reduced organic matter often have strong hydrogen sulfide odors signifying a sulfur-reducing bacterial presence. This process also occurs under salt marshes and explains why sediments under them often contain high aluminum levels. A by-product of this process is the common sulfur smells. Since dissolved hydrogen sulfide gases from creeks and salt ponds are toxic to most fish species and most harmful in warm water which can hold less oxygen. This sulfur reducing process also explains why eelgrass meadows frequently show extremely high sulfide levels below them as its ability to slow surface water flows and trap organics, helping to separate these two nitrogen/respiration pathways. High sulfide levels are toxic to most marine organisms. In fact, in the aquarium and aquaculture industries, the cause of “black death” or “black water death” is from the sulfides found in them. Changing filter systems in the first commercial bio filters have been dangerous since the first closed system aquaculture operations were constructed. This gas releases when these sediments “boil” even at low temperatures can cause killer toxic gas events in the tropics near large lakes with high organic matter inputs.
Removing sulfide-rich deposits to oxygen sufficient areas as dredged material allows the oxygen-nitrogen pathway to continue producing nitrates, a plant nutrient that favors vascular plants (submerged aquatic vegetation). The nitrogen-sulfide pathway produces nutrients that favors plankton especially the browns that so devastated eastern Long Island’s Peconic Bay scallop fisheries in the 1990s. High heat drives the nitrogen-reducing pathways from the oxygen sufficient towards the oxygen deficient sulfur reduction process. Brown plankton blooms often occur during periods of high heat and low energy because of the enormous supply of ammonium and reverse with green algae in cooler and energy prevalent periods. This happened during The Great Heat of 1880-1920 and from Connecticut’s coastal core studies many times before.
Closed system aquaculturists have long realized how important oxygen sufficient, nitrogen-reducing bacteria are to the ammonium to nitrate cycle for fish culture. Home aquariums also are subject to the some habitat failure when filters are overwhelmed with organic matter and turn black. Submerged aquatic vegetation that traps organic matter in high heat can accelerate this habitat degradation process. Eelgrass meadows in high heat have been known to produce extremely high sulfide levels beneath them. Having oxygen-reducing bacteria shift to oxygen-deficient sulfur reduction kills bio filters and ammonium levels soar. In the marine environment, this occurs on a massive system-wide scale especially in shallow, warm, poorly flushed coves and bays. Sulfate-reducing bacteria combined with high heat shift the balance to plankton, not vascular plants providing the ready access “fuel” needed to sustain these intense algal blooms (HABs) associated with high heat habitat reversals. These habitat reversals can be decades of more in duration as banked organic sulfur-rich deposits build-up and can be a nitrogen source for centuries. This situation is also described by Hynes (1971) in his lake studies.
“In an oligotrophic lake there is little oxygen demand in the hypolimnion because of the general paucity of life and the absence of much organic matter sinking from above. The store of oxygen is therefore sufficient to last until the autumn, when complete mixing again occurs because of the cooling of the epilimnion. In a eutrophic lake on the other hand there is a large oxygen demand in the hypolimnion because of the constant rain of dead and dying plankton, and all the oxygen is used up during the summer at least near the bottom. This is of course has marked effects on the benthic fauna, which do not concern us here, but it also affects the release of nutrients from the dead organisms. Under aerobic conditions these salts tend to remain in the mud, and relatively small amount of them find their way back into the water; under anaerobic conditions, however, they are released very rapidly into solution and hence, ultimately, back into the biological cycle.
Therefore, as a lake reaches that state of productivity which results in total de-oxygenation at the bottom of the hypolimnion it becomes considerably more productive, and may begin to produce plankton blooms quite suddenly. It is at this stage that the general public becomes aware that the lake has changed, and within a very few years there may be marked losses of amenity.”
Dredging, therefore, has the ability to remove this nitrogen bank that could take decades or longer to naturally decompose and restore previous tidal flows, and in times of high heat, mitigate high heat habitat failures. This improvement in water flows promotes oxygen reduction processes and not one that supports a sulfur-reducing pathway.
That is why fishers often report increases in fish abundance following dredging projects, especially those that expose glacial sands and cobbles to the tidal fluctuations. Such areas have been shown to carry a limited, cool ground water oxygen reserve for the smallest winter flounder. Dredging removes acidic compost and by doing so, reverses soil acidity. Post-dredging surveys of sands rinsed of organic acids often show increased sets of bivalves (temperature dependent Paul Galtsoff, USFWS 1964). Bays and coves with reduced flushing often show the build-up of sulfurous mucks and soils. We need to look at dredging in a new light, not always the negative but a process that could turn back the habitat “clock” for some fish and shellfish species, reduce the build-up of nitrogen, and shorten periods of anoxic conditions in coves, bays and sounds and marine life in these degraded habitats will rebound. As a public policy viewpoint not all dredging should be considered as “bad.”
The 1870s and 1950s were two periods of cold winters and numerous storms (increased energy pathways). Reports from fishers frequently mentioned the presence of firm harbor bottoms and a firm sand/estuarine bivalve shell matrix which soon became a dominant habitat type. Organic matter banking and nitrogen enrichment of composting material did not occur. It simply was washed away by storms and the oxygen sufficient, bacterial reduction processes dominated sediment respiration. This was not the case during The Great Heat, a cycle of increased heat and few storms that occurred from 1880 to 1920. That period resembles almost precisely the period from 1974 to 2004. Historical fish and shellfish records make mention of increased smells from marshes (rotten egg and methane smells) and changes in bay and cove bottom firmness (habitat types). Numerous accounts from Cape Cod to New York’s Peconic Bay Long Island Sound, Rhode Island and Connecticut refer to deep accumulations of organic matter, a black, jelly-like material that seemed to increase in depth. This increase can be quite rapid and can take the public by surprise as mentioned by H.B.N. Hynes in his 1971 book The Biology of Polluted Waters from his studies of lakes.
“It appears that about half the nitrogen is built up into organic matter in these lakes and that there is also adequate phosphate for this enormous amount of plant growth, the wet weight of which would be at least 100 times as much as the amount of nitrogen used. Even if nutrient salts are added while still bound up in organic matter they become rapidly available for algal growth (Flaigg and Reid, 1954; Ohle, 1955), so it makes little difference if they are added as purified or unpurified effluents, although of course ordinary biological treatment does remove some saline nitrogen and phosphate by sedimentation. Ohle (1955) states the raw sewage sometimes contains as much as 15 mg/1 of phosphate phosphorus, but treated effluents contain usually only 2-4mg/1. although as much as 6-8 mg./1. may remain.
In a recent study of a large lake near Copenhagen (Berg et al., 1958) it has been calculated that, because of pollution, about 24 tons of saline nitrogen and 4 tons of saline phosphorus enter the water each year, and that this represents about 12 per cent of the total amount used by the plankton. Moreover very little of this nitrogen and phosphorus leaves the lake via the outflow, the calculated amount being about 3 1/2 tons of nitrogen and 200 lb of phosphorus. This emphasizes the fact that lakes are very efficient traps of fertility, and that even slight pollution is likely to cause a rapid increase in the rate of ageing.
Unfortunately the change seems to be irreversible – once a lake has become eutrophic it remains so, at any rate for a very long time, even if the source of extra nutrients is cut off (Hasler, 1947). Another unfortunate feature is that the onset of extreme eutrophy appears to be a rather sudden feature in lake development, which takes only a few years to become manifest. Its appearance therefore tends to take the general public by surprise.”
This change in habitat type, from hard to soft, was noted as declining or degraded habitat conditions for bay scallops, hard clams, oysters and winter flounder, while increasing habitat conditions for the blue crab, green crab and soft shell clams. However, in areas with slow tidal movement or poor “flushing,” large fish and shellfish kills were often reported, signallying extended periods of oxygen deficiency or anoxia. This cycle seems to reverse physical habitat characteristics but also chemical/bacterial ones as well. It is known that the movement by storms or dredging of deep organic accumulations into oxygen sufficient waters lowers the populations of sulfate-reducing bacteria and the oxygen-reducing bacteria soon increase.
In dredged material disposal sites that have good tidal exchanges, waves, currents and tides (energy pathways), organic matter quickly reenters the marine food web, it is fish food. However, such deposits in oxygen-poor waters contribute to the production of ammonium ions, making nitrogen subject to the same energy and temperature cycles creating a direct habitat quality link. This link introduces a weakness in the nitrogen abatement models in many estuaries today as its primary focus is upon human nitrogen inputs while minimizing the role of organic source nitrogen. That is a large mistake in warm poorly flushed coves and bays. Therefore “black mayonnaise Sapropels form first in the “back waters” and advance into the headwaters. This is quite noticeable in the upper reaches of the Mystic and Poquonnock Rivers bisected by railroad causeways.
One of the largest problems with the use of nitrogen as a marine pollution indicator is that is also is subject in the marine realm to wide swings of temperature and energy, the key factor being oxygen. Nitrogen compounds entering Long Island Sounds as dissolved organics generally are not subject to the nitrogen-sulfur reduction process, a huge distinction in times of few storms and high heat.
Most of the nitrogen cycle information is based upon the terrestrial model. In this model, bacteria in the presence of oxygen (our atmosphere) converts ammonia NH3) to an ammonium ion (NH4) which then undergoes a further process converting nitrite (NO2) to nitrate (NO3), a plant nutrient.
In the presence of oxygen and adequate mixing (high energy), the bacterial, nitrogen-fixing process favors ammonium ion in water while supporting two types of bacteria, nitrifying and denitrifying bacteria which as end products release methane gas into the atmosphere and available nitrate compounds. When Sapropel forms it usually signifies a shortage of nitrate as sulfur bacteria switch to utilize sulfate instead.
However, in oxygen-limited waters, especially during periods of high heat and insufficient mixing (low energy), another nitrogen pathway exists, mostly in waters that are warm and receive large amounts of organic rain (sometimes referred to as marine snow). In this case, high amounts of crushed wood debris, leaves and stems found on street surfaces enter water bodies as an organic slurry during heavy rains. In some organic, high sulfur mucks, 50% of the material can consist of leaves and stems (personal observations). In commercial and recreational shellfishermen accounts, this material is called “oatmeal,” and in some cove and bay bottoms, can be feet deep and brown in color. West of the Guilford, Connecticut region, this “oatmeal” at times can contain fragments of stem material from phragmites species. It is this “oatmeal” that during high heat stimulates the sulfur-reducing bacteria in the absence of oxygen. Its reappearance in coastal waters is attributed to these factors.
1) Organic inputs such as leaves, woody debris and dead grasses from poor watershed practices can overwhelm coastal reduction processes.
2) This detrital debris is not washed from poorly flushed areas due to reduced energy pathways tidal restrictions and actually accumulates in high heat periods.
3) High heat reduces the availability of oxygen to complete the nitrogen cycle, favoring a nitrogen-sulfur reduction process.
It is this organic material that “cooks” in the marine environment and is most damaging to coastal marine habitats. While dissolved nitrogen compounds can move with the tides be attenuated (often before reaching Long Island Sound) impacts should be seasonally adjusted for temperature. Cold winter temperatures drive the reduction processes back to oxygen bacterial from sulfur bacterial processes. Colder water contains more oxygen; that is why some fishers’ accounts mention several feet of “oatmeal” in the fall only to return in the spring to see this material absent. (It was reduced and moved by winter storms.) These accounts also mention that when an area is dredged, the remaining sulfide rich organic matter seems to “melt away.”
When examining the habitat quality factors, organic matter nitrogen is much more damaging than dissolved nitrogen compounds or “people nitrogen.” It is known that sulfur-reduction processes can lower ambient pH, produces sulfuric acids that can destroy concrete bridge abutments, can lower the pH in marine soils thus preventing bivalve (shellfish) sets, can drive oxygen levels lower, and can sustain longer periods of anoxic conditions. In the 1950s, during a period of colder temperatures and incredible energy (large number of storms), Long Island Sound was at times, found to have nitrogen limited and anoxic conditions were few and of short duration.
Finally, one of the largest habitat factors identified to date is that marine organic compost tends to produce ammonium, an ion that is needed by harmful algal blooms (HABs). That is why HABs are often occur late in the summer and are densest in poorly flushed bays and coves where ammonium ion concentrations can reach high levels. High ammonium levels are needed to quickly sustain such large and intense “blooms.” HABs during the 1950s, were practically unknown to Long Island Sound waters and New York bays.
Hydrogen sulfide reduction is easily seen in the marine environment, the color of salt marsh banks, the infamous odors of black, partially reduced mucks, Even the reduction of sulfate ions (SO4) can be seen by the casual beach walker; it is responsible for the blackening of the undersides of beach cobblestones sealed from the oxygen above and when turned over has a black stain.
The reduction of organic matter by sulfur-reducing bacteria is extremely slow, much slower than oxygen-reducing bacteria. That is why terrestrial composters will regularly “turn” compost piles to mix them with air/oxygen. In the marine environment, high sulfide levels contribute to low pH soils and can degrade habitat quality for both fish and shellfish. Nitrogen compounds are then banked as mentioned previously into this black material now rich in metal sulfides.
SO4 plus sulfate-reducing bacteria plus organic matter yields H2S gases (rotten egg smell)
The sulfate-reducing bacteria and sulfur-reducing groups only tells part of the story, anaerobic bacteria break down (reduce) some of the phosphorus and nitrogen compounds locked away in plant tissue, especially leaves (due to the increase in forest canopy). While nitrogen is “fluid,” (aqueous) it can quickly travel taken by tides and currents to oxygen sufficient areas. Organic matter however, does not share this mobility; when it reaches estuaries, it tends to collect in bays and coves, poorly flushed areas.
Fishermen in eastern Connecticut in the early 1980s complained bitterly to state officials claiming a “Tampa Bay effect” by the shore/coastal railway that bisected many eastern Connecticut coves. With tidal exchange reduced, residents, many of whom were shell and fin fishers, noticed a build-up of sulfurous muck in areas that once contained many shellfish and finfish species. In some cases, three feet or more covered oyster beds. (Visel, DeGoursey, Auster 1990) This material, organic matter or marine compost, “cooks” or reduces in high heat. Anaerobic bacteria with organic matter produces a nitrous oxide, a gas, and results in the brown coloration of material. However, in high heat, this material can turn black signifying high sulfate levels and decomposes into sapropel, a blue/black substance rich in hydrogen sulfide and methane. These are the gas bubbles that can be seen rising from these deposits, especially in Hamburg Cove, Lyme, and Middle and North Coves in Essex, Connecticut. On a spring day, when the water is very cool and clear, you can watch these gases venting from these soft sticky deposits. These areas are usually devoid of fish life with the little benthic relief. Look for this sapropel in Connecticut’s poorly flushed coves or those with severe today restrictions which acts more like a dam and lake conditions described in the front of this report.
Thus, in terms of nitrogen residence time or bank, these reserves of nitrogen containing compounds can last for decades or centuries depending upon temperatures and energy levels. That is why linking the reduction of human nitrogen inputs to a return of fish and shellfish species is somewhat misleading, or false if not indexed for temperature or energy levels. When the two nitrogen reduced pathways are compared, the sulfur pathway is much more damaging to marine ecosystems and largely out of our control (temperature). However, we can alter the energy pathways; that is where dredging comes in It is just moved from oxygen in sufficient to oxygen sufficient areas such as dredge material disposal sites. While organic nitrogen enters water columns in two forms, ammonia oxygen-reduced suitable for broadleaf plants and ammonium from bacterial denitrification. It is the ammonium ion that is quickly utilized by the brown algal species. In high heat and low energy conditions, high concentrations of the ammonium ions can sustain damaging HABs, harmful algae blooms as the bay scallop fishermen in eastern Long Island will recall in the 1990s. Extreme heat and low oxygen altered the dynamics of the nitrogen cycle, blocked to some extent by the rates of nitrifying bacteria nitrosomonas and the opening the sulfur-reduction process to lower pH and facilitating anaerobic bacterial processes, thereby increasing the proportion of ammonium to ammonia levels. In other words, the “nitrogen problem” is not so much an input problem but one related to climate and temperature. Therefore, historically the brown algae species did so well in the 1880-1920 hot period and the 1990s and why green algae biology predominated during the colder and more energy prevalent 1870s and 1950s.
During cold periods – human inorganic nitrogen inputs (ammonia) have more impacts than terrestrial sources. In times of great heat however the “banking” impacts of nitrogen phosphorous containing (leaves woody, debris, dead grass vegetation) make human aqueous nitrogen (easily moved by tides and currents) inputs appear minor in comparison. Thus dredging can reduce the amount of extent of low pH sulfide rich accumulations and increase ambient oxygen levels necessary for aerobic bacterial respiration of organics similar to the process in modern wastewater treatment plants.
Dredging marine areas can speed the recovery of nutrient enhanced environment (such as what currently happens with lakes and ponds) as many studies today link nutrient enhancement to diminished social and economic values. Maintaining suitable open water disposal areas is key to allowing this process to happen. Closing the dredge disposal sites is the equivalent of closing composting facilities. Only here the component is fish food.
Having one or more active dredged material disposal sites will not only continue the critical economic benefits from maritime commerce, the boating and navigation interests (marinas) including jobs and related dependent businesses but can help remove banked nitrogen.
Summary –
The principal harm to Long Island Sound’s Fisheries – the ones that presently have value is a lack of energy and an increase in temperatures. The principal harm to Connecticut near coastal habitats has been the increase in paved surfaces and the tremendous increase in Connecticut’s forest cover – leaves as organic matter inputs. In cycles of high heat and low energy tidal flushing in coves, bays and lower rivers depths are reduced. Organic matter collects lessens estuarine pH and becomes a composting high sulfur habitat. Acidic high sulfur environments are some of the most damaging to oxygen dependent species. Moving this organic matter into oxygen sufficient areas releases bound up organic matter back into the marine food chain (fish food) removes a nitrogen sink and ends disease producing sulfur bacteria strains.
To maintain energy pathways and maintain navigation during this warm climate cycle it is essential therefore that dredged material disposal sites remain open. In fact to handle organic debris (leaves, wood, rot, etc) other sites should be created. Increasing hydraulic capacity such as man made salt ponds deepening salt water access could in fact reduce hydraulic stress – flooding during severe storms. It could also add habitat refugia for the blue crab whose populations now cling to a predator free habitat zone in dredged marina basins and channels presently.
Dredging marine composts to enhance habitat quality may have a precedent, in New York late 1970s, conversations with Peconic Bay Fishers years ago told of dredging accumulated duck farm feces from coves. I plan to investigate this incident later this spring. It was the small boat commercial fishers (baymen) from Great South Bay and Peconic Bay, New York, The South County Rhode Island Salt Ponds, Pleasant Bay on Cape Cod and Niantic Bay in Connecticut were the first ones and report the build up of sapropel – the hydrogen sulfide mucks. This build up continues along Connecticut’s coves and river systems. Some of the deepest deposits I have observed in recent years has been Hamburg Cove – Lyme and North, Middle and South Coves in Essex. Middle Cove Essex has most likely 8 to 10 feet, Hamburg 12 to 15 feet (mostly leaves) North Cove Old Saybrook has a dredged mooring basin which sapropel is removed and has become an important habitat refuge for the blue crab. The gas venting from sapropel in Middle Cove Essex in spring is the heaviest I have ever observed and reported by kayakers in these coves.
It is important to keep disposal sites open for the boating industry but also to investigate habitat mitigation and nitrogen reduction projects. Dredging can be a nitrogen reduction and habitat restoring activity. Much of the organic matter now accumulating in coves has tilted the balance to the sulfur cycle (especially in times of high heat) and should be part of new study.
I hope these comments will be a help to the EPA Scoping Document process as a
supplemental impact statement.
Comments submitted to Alicia Morrison – Grimaldi
Ocean and Coast Protection
Environmental Protection Agency Region I
Boston, MA
This comments and views are my own reflection of four decades of working with the boating and fishing industries. They did not reflect the view or position of either the
Citizen’s Advisory Comment or Habitat Restoration Working Group of the EPA Long Island Sound Study of which I presently belong.
By Timothy Visel
For printed quotations
The biology of polluted waters by H.B.N. Hynes Professor of Biology – University of Waterloo, Ontario, Canada with introduction by F.T.K. Chief Inspector of Salmon and Freshwater Fisheries Ministry of Agriculture Fisheries and Food, London England - University of Toronto Press 1971.
Appendixes -
Appendix (1)
The Impact of Energy – Tidal Exchange as Referenced by Inlet Width and Hard Shell Clam Production NOAA Publication (Marine Fisheries Review Vol 64, No. 2, Clyde L. MacKenzie, Jr., et al 2002.
Appendix (2)
Sapropel Buildup North of the Pattaquansett River Railroad Bridge East Lyme, CT USA Published Abstract April 5, 1990 – Visel – DeGoursey – Auster, University of Connecticut.
Appendix (3)
Sapropel Builtup Middle and North Basins Poquonnock River – above Railroad Crossing – Report to the Groton Shellfish Commission – Tim Visel, June 1985.
Appendix (4)
The Consequences Of Insufficient, Tidal Flushing – 1974
Tidal Wetlands of Connecticut, Niering/Warren, Steever
Marine Fisheries
Review Vol. 64, No. 2
2002
Excerpt by:
Clyde L. MacKenzie., Jr., Allan Morrison, David L. Taylor, Victor G. Burrell, Jr.,
William S. Arnold, and Armando T. Wakida-Kusunoki
Quahogs in Eastern North America; Part 1, Biology, Ecology, and Historical Uses
Page 8 Large Bay and Ocean Water Exchange Attributes
In the northeastern United States from Massachusetts through New Jersey, the bays that have a large exchange of their waters with ocean waters now have relatively large stocks of northern quahogs, while those with poor exchanges have small quahog stocks. The areas with large exchange are Buzzards Bay, mass.; Greenwich Bay and Point Judith Pond, R.I.; Long Island Sound, Conn.; and Raritan Bay, N.Y. and N.J.. The bays were the exchange is poor are Great South Bay, N.Y., and new Jersey’s coastal bays (Barnegat bay, Little Egg Harbor, and Great Bay). The water in the zones of Great South Bay farthest from the bay inlets exchanges with ocean water only once every several weeks (Nuzzi).
Great South Bay once had large stocks of quahogs, McHugh (1991) reported the opening of an inlet between the Atlantic Ocean and Moriches Bay (which connects with Great South Bay) on Long Island, N.Y., made by a hurricane in 1931, led to a large increase in salinity in Great South Bay. The higher salinity allowed oyster drills to increase in abundance and activity, and they substantially reduced the numbers of remaining oyster (MSX might have also been responsible, (Usinger), but dense quahog sets occurred throughout the bay and a substantial quahog fishery developed. Moriches Inlet eventually closed, but a hurricane in 1953 reopened it. By 1957 it began to close again. In 1958 it was widened and deepened by dredging and subsequently protected by a seawall. Jeffrey Kassner believes this 1958 opening may have set the environmental state for the boom in quahog production in Great South Bay in the 1960’s and 1970’s.
Ingersoll (1877), who surveyed the mollusk fisheries in 1877-78, reported that Barnegat Bay was called “Clam Bay” and yielded 150,000 bushels of quahogs/year. The area now yields barely 1,000 bushels of quahogs/year. Charts from 1878 (Woolman and Rose, 1878) and 1997 (NOAA Nautical chart 12324) show the amount of housing on the shores, the bay itself, the location of Barnegat lighthouse (wide, open arrows on both charts), and widths of the inlets (Fig.12). Little housing is shown in the 1878 chart, but a considerable amount of housing is suggested by the canalization of the shorelines shown in the 1997 chart (houses crowd the shores of all canals). The buildup of housing took place in the 1960’s and 1970’s (Collins and Russell, 1988). The width of Barnegat Inlet in 1878 was 4 times its width in 1997. There likely was considerable exchange of bay and ocean waters and little eutrophication of bay waters in the 1870’s. This contrasts with limited water exchange and considerable eutrophication of bay waters in the late 1990’s.
Inlets that have been opened by hurricanes seem to have had beneficial effects on quahog populations in North Carolina. Chestnut (1951) stated an increased quahog abundance in northern Core Sound during the mid-1930’s appeared to be associated with the opening of Drum Inlet by a 1933 hurricane. Godwin et al, (1971) reported a similar occurrence related to Hurricane Hazel in 1954. Hurricanes do not exert negative effects on quahogs in North Carolina, although the closing of an inlet by a storm has a negative effect. When any North Carolina inlets closed, nearby quahog stocks declined (Taylor, 1995).
Reduced Oyster Recruitment in a River With Restricted Tidal Flushing
Timothy C. Visel
Sea Grant Marine Advisory Program
The University of Connecticut at Avery Point, Groton, CT 06340
Robert E. DeGoursey, Marine Sciences Institute
The University of Connecticut at Avery Point, Groton, CT 06340
Peter J. Auster, National Undersea Research Center
The University of Connecticut at Avery Point, Groton, CT 06340
The Pataguanset River in East Lyme, Connecticut, historically supported a natural oyster bed that has recently declined in productivity. A series of surveys of the river (1985-1988) identified one natural bed comprised of large adult oysters (10 cm to 18.7 cm shell ht.) and few juveniles (<4.6 cm shell ht). The reintroduction of an oyster fishery would quickly deplete this resource without substantial recruitment of seed oysters. Three attempts to restore the oyster setting capacity of the bed by cultch planting and shell base cultivation were unsuccessful. No new seed oysters were observed. Direct underwater observations confirmed heavy silting of newly planted shell cultch, preventing the setting of oysters. Further examination of the lower Pataguanset River near a railroad causeway revealed a historic oyster bed buried under approximately 1 meter of organic sediment. The construction of the railroad causeway reduced the overall width of the river from over 1,000 meters to approximately 15 meters. Effects of the causeway including increased siltation and reduced salinities due to restricted tidal flushing, have negatively impacted the population dynamics of the natural beds. Ideally, tidal flow should be restored. However, management under the current hydrologic regime should include hydraulic cultivation and intensive shell base maintenance in order to enhance oyster productivity.
National Shellfisheries Association, Williamburg, Virginia Abstracts,1990 Annual Meeting, April 5, 1990 – pg 459.
The Day, New London, Conn., Wednesday, June 12, 1985
Specialist warns agency of ‘black mayonnaise’ threat
By William Hanrahan
Day Staff Writer
GROTON – they call it black mayonnaise – it’s the murk and muck, sometimes several feet deep, that collects on river bottoms. It’s also the stuff stifling the area’s oyster crops, according to an expert.
Addressing the town’s Shellfish Commission Tuesday night, Timothy c. Visel, a marine resource specialist for the University of Connecticut, said the build-up of debris in shellfish area’s can weaken or eliminate growth.
Working in waters off Old Saybrook, Clinton and Madison, Visel said production of oysters there has more than quadrupled thanks to clean-up efforts during the past three years.
“There seems to be a trend that our rivers are filling up with black mayonnaise,” he said. “We have seen a dramatic increase in river life as the dead stuff is removed.”
The accumulation of debris occurs in waters with poor circulation. “We get so many nutrients going into these sluggish coves without a lot of circulation,” Visel said. “This causes a build-up and no oxygen gets down in the water.”
Visel said removing debris not only enhances oyster growth, but has increased the presence of a number of other fish, including flounder.
Visel said Connecticut used to be a leader in oystering about 100 years ago, with local areas such as the Poquonnock River as prominent beds. More than 100 oyster companies on Cape Cod used to rely on seed oysters from Connecticut which were brought there to mature.
Production dwindled to almost nothing as waters became polluted, he said. A clean water act in the late 1960’s helped rekindle the industry during the 1970’s, but things are still not what they used to be.
Removing black mayonnaise |
|
Back to top |
|
|
|
|
You cannot post new topics in this forum You cannot reply to topics in this forum You cannot edit your posts in this forum You cannot delete your posts in this forum You cannot vote in polls in this forum
|
|