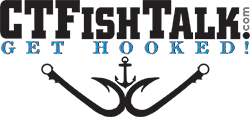 |
|
View previous topic :: View next topic |
Author |
Message |
BlueChip
Joined: 29 Jun 2011 Posts: 177 Location: New Haven/Madison/Essex
|
Posted: Wed May 16, 2018 10:14 am Post subject: Marine Soil Bacteria and Water Temperature EC #16 |
|
|
Marine Soil Bacteria and Water Temperature
Environment/Conservation #16
Ultraviolet Light and Aquaculture Ecosystem Services
Bacteria and Nitrogen As Habitat Quality Indicators
An Estuary Monitoring Capstone Proposal
FFA SAE – ISSP Capstone Research and Discussion
Updated to April 18, 2018
The Sound School
60 South Water Street
New Haven, CT 06519
View All Bacterial Nitrogen EC Newsletters on the Blue Crab Forum™ (Environment Conservation Thread) and
FishTalk™ – Saltwater Reports
The views expressed here do not reflect the Citizens Advisory Committee or Habitat Stewardship Working Group of the EPA Long Island Sound Study. On February 16, 2016, and February 8, 2017, I have asked Connecticut resource management agencies to recognize Sapropel as a distinct subtidal habitat type. This is the viewpoint of Tim Visel.
Environmental and Conservation Thread #13 Blue Crabs, Salt Marshes and Habitat Succession was posted on July 13, 2016, and should provide an introduction to this habitat history concept. It has been one of the most viewed posts to date.
An Update from Tim Visel, April 2018
Marine Soil Bacteria
This report was started in 2016, and since that time, more information has come forward in the research literature about eelgrass and soil bacterial biochemistry. The formation of sapropel, a low oxygen sulfidic organic compost, is now linked to eelgrass root failure. Eelgrass, when subjected to heat, endures a stressful soil change, from a soil that maintains oxygen bacteria to one dominated by sulfate bacteria (Microbiome Succession During Ammonification In Eelgrass Bed Sediments by Eltinger et al., 2017). Plants need beneficial oxygen bacteria surrounding its roots, and eelgrass has the ability, when subjected to sulfur metabolism, to move oxygen from leaf tissue to its roots, effectively maintaining its rhizome root bacterial shield from sulfide intrusion. Sulfides in marine soils (especially in warm or hot marine deposits) contain higher sulfide levels (See “Relationship of Sediment Sulfide to Mortality of Thalassia testudinum In Florida” by P. Carlson, L. Yarbro, & T. Barber, 1994 and “Sulfide Intrusion in Seagrasses Assessed by Stable Sulfur Isotopes: A Synthesis of Current Results” by Holmer & Sheetal, 2014), which act to destroy eelgrass beneficial root rhizome shield of oxygen preferring bacteria – the bacteria that assist the transfer of nutrient ions across this shield into root tissue. Lose this bacterial shield and the eelgrass plant will eventually die. To prevent this rhizosphere collapse, eelgrass has an unusual capacity to move oxygen from (leaves) plant respiration to its roots, “leaking” oxygen from root tissue to help keep its helpful bacteria alive with a process called “ecological engineering.” This ability to move oxygen from leaf tissue to roots is limited if metal ions and sulfide become too dense, then the rhizosphere shield collapses and roots begin to rot. This movement of oxygen also weakens the plant and leaves it “open” to fungus and biofilm damage, reducing oxygen exchanges. This is the brown and furry (slimy) eelgrass that is mentioned in the eelgrass literature. This process in heat and a growing organic humus is the cycle of sapropel; and if long enough, it will kill eelgrass. What is not mentioned is the “bacterial war” beneath the eelgrass growths between bacterial groups, those that utilize oxygen and those that do not, turning to sulfate and “wasting” sulfide, a plant (eelgrass) toxin, into the soil. What can alter this situation is cooler seawater (more elemental oxygen) and aeration of soil itself – natural cultivation events – storms. It is natural after cold or cooler periods with storms to see eelgrass coverage expand. It is also natural that in periods of low storm activity and very warm seawater, eelgrass will “dieoff” or coverage lessens and constricts. The cycles of sapropel is connected to cycles of eelgrass.
Sapropel is the continued bacterial decomposition processes with very low or no oxygen present. The remains of decades of leaves, dead grass forest litter now rot, producing a sulfide-rich loose ooze. During floods, these deposits are ripped up and deposited downstream. Brown water from suspected leaf tannins after Hurricane Agnes (June, 1972) carried, it is thought, enormous amounts of tannins, a brown natural flocculent and the remains of anoxic leaf paste, a brown or “chocolate water” event. The tannins are acidic and capable of staining, and witness accounts provide clues to them. They turn things brown or “rusty.” They also can aerate sapropels and give rise to the sulfide rotten egg smells. However, sapropels are dislodged by flooding waters and this witness account contains both observations – the sulfide/tannin browning and the smell – from the Times leader by Bill O’Boyle, June 18, 2017:
“Forty-five years later, Agnes still on people’s minds Wilkes-Barre City Councilman Tony Brooks recalls the event. Brooks noticed the change in the color of the grass, the sidewalls and the streets, everything touched by the river was now brown … and then there was that smell, - It was such an awful smell to this day – regardless of your age, you can remember the smell of flood mud.”
These events leave lasting memories of the damage and human loss but below the water also have lasting impacts to shallow water habitats, including those for the blue crab. The flow rate at the Conowingo Dam during Agnes was one million, one hundred and thirty thousand cubic feet of water per second (Taylor K.R., 1972) as reported “The Effects of Hurricane Agnes on the Environment and Organisms of Chesapeake Bay – A Report to the Philadelphia District U.S. Army Corps and Engineers prepared by the Chesapeake Bay Research Council, January 1973 (Andersen, Davis, Lynch, & Schubel) VIMS #29 (180 pages).
One of the factors associated with tannin/sapropel movement is the release of zinc followed by aluminum. This is the result of sulfate acid soil (sapropel) being uncovered and aerated, resulting in a sulfuric acid release accompanied by tannin release, the so called “chocolate waters.” Zinc increases were explained as that “zinc desorbes from the freshly deposited sediment (now suspected of containing sapropel) as a result of cation exchange after the riverflow subsides” (pg. 71), noting also increases in copper. These factors support the event as a huge tannin/sapropel release, tannin from natural leaf breakdown and sapropel deposits exposed once again to oxygen, generating a sulfuric acid “flash” to release metal ions. Sewage, leaf matter and heavier wood debris were swept downstream and now subject to bacterial action following the storm event.
And now four decades after Hurricane Agnes, some answers to many questions about the sudden drop of the young of year index of striped bass. The sulfuric acid release of aluminum ions bound in clay and organic particles creates perhaps highly toxic conditions for the striped bass.
Marine Composts and the Release of Aluminum
In times of heat and low water flows, habitats become warm in shallows. Peat collects heat and these habitats can become hot and sulfide-rich. The loss of shallow water habitat now has a sapropel/aluminum link. Aluminum as a free ion is highly toxic to fish; it is a positive charge ion and damages oxygen exchange capacity in gill tissue.
In an environmental state, aluminum is held by the negative charge of clay particles. In this form, it is non-toxic (non-mobile), but in acid waters or waters that overturn humus, sapropel that create very low pH conditions and now aluminum is “mobilized.” That is, it is now released from the clay particles. This occurs after large rainfalls or extreme heat. In this instance, a possible Hurricane Agnes connection to the rapid decline of the young of the year striped bass nursery habitats, post-event into low energy areas. Some of the reasons for the sapropel/aluminum connection, in fact, have been mentioned in the first striped bass press accounts. Sapropel from organic matter swept into shallow water by strong storms can take years to develop. A 1985 New York Times article refers to this low pH impact, but it is the mention of aluminum and acidity that provides some clues. Nelson Bryant states (more than a decade after Hurricane Agnes):
“Some of the acidity in stream, lake or pond comes from natural, organic acids. It is also known that the interaction of acidic water with miniscule amounts of aluminum, a common trace element in soils containing much clay – increases a stream’s toxicity to the larvae.”
Agnes’ impact may have many factors – sweeping large amounts of accumulated clays - with aluminum in bay waters, also moving huge amounts of organic matter, manure, sewage, forest duff, grass, bark and leaves into the watershed and finally moving sapropel deposits downstream as well.
Sapropel may form over the summer in heat and low oxygen conditions and then in spring rains with storms move sapropel and, in doing so, create acidic (sulfuric acid) and clays carried into estuaries would also bring aluminum. This may continue far beyond the storm event. This leads to a single event as existing habitats (over time) perhaps transition into these more favorably. As habitats for small striped bass failed in the Chesapeake – those associated in New York, even Connecticut, improved. As habitats declined to our south, the importance of the Hudson River striped bass habitats in the north increased as reported in the fisheries literature.
A look back, the aluminum connection becomes an important issue as a large spawning striped bass population existed at the time of Agnes (catches were high in 1973). It looks like a habitat failure although most later press accounts looked primarily at overfishing as the cause, but that would take time as well, and makes the habitat connection to sapropel formation at times difficult. The “habitat clock” was reset by Agnes but its habitat connection was delayed; however, not so for the blue crab. After Agnes came several negative reports, but blue crabs can rebuild quickly and the loss of a serious apex predator, the striped bass actually could help blue crab recruitment.
Introduction
For decades, estuarine researchers were aware of bacterial shifts in “sediments,” a term that has been a catch all for most of the nitrogen and bacterial chemistry reactions in marine soils. The bacterial association of agriculture soils has been documented for over a century – the uptake of nitrate as a plant nutrient.
Shellfish depend also upon the creation of nitrate from this bacterial process to feed algae, its food and nitrate, at times, can become limiting in very cold conditions, which reduces bacteria growth. When that happens in agriculture soils, we often add nitrate so that plant growth and crops are not diminished.
In heat, these marine soils reverse the nitrogen pathway as they do in terrestrial soils and depend upon the type of bacteria that are present – in cold, oxygen bacteria; in heat, the bacteria that utilize sulfate to “breathe” (produce ammonia). It is the sulfate-reducing bacteria that cause so much damage to oxygen-requiring life.
Although nitrogen compounds have been under scrutiny for negative watershed impacts (over-enrichment water contamination), the role of nitrate buffering or the ability to augment low oxygen conditions has not. Nitrate in seawater fertilizers the algal food for shellfish, which then filter the water and in a way controls bacteria growth. In times of heat and low energy, nitrate becomes an important oxygen source for those bacteria that reduce ammonia (highly toxic) to beneficial and less toxic nitrite and nitrate compounds, which each contains “oxygen” in bonds that the good bacteria can break. In high heat/low elemental oxygen conditions, the availability of nitrate helps keep nature’s bacterial filters alive in sands and surface bacterial films, much as in modern aquaria and aquaculture systems.
Just before this natural filter fails, nitrate levels drop and ammonia levels quickly rise. High ammonia levels favor different types of algal populations that need it and are not favorable for shellfish. The Browns or HAB’s studies now show that high ammonia levels shield the HAB species spore (cysts) as well as keeping oxygen bacteria at low numbers. Waters that were nitrate rich in cold become ammonia rich in heat.
This filter reversal has been designated as a “benthic flux” but its role in nitrogen generation (ammonia) and sulfate metabolism often remains unreported in the current literature. It has a direct temperature link and values nitrate as buffering low oxygen conditions, something that waste water treatment facilities used for much larger filter systems since the 1980’s. Cloudy or turbid water contains particles for bacterial growth. They also prevent ultraviolet light penetration, and in heat, foster the growth of sulfur bacteria, not oxygen bacteria. Cold waters are typically clear, thus linking water temperature to bacterial change and densities as ultraviolet light can penetrate deeper into the water column.
Temperature Changes And Nature’s Bacterial Filters
Although Maine near shore waters have documented a 1oF drop in coastal water temperature (Working Waterfront Issue December 2017), Long Island Sound has also cooled; three cold/snowy winters and three cooler springs has reduced the summer peaks from 81oF to 73oF at Essex, CT town dock. This change we more than experience in one day, a cool morning followed by a warm afternoon can easily show a 25oF change, but water takes much longer to heat up and cool down, sometimes years. However, these differences in water temperature can result in huge changes for fish and shellfish. The slightest change in degrees, over time, can in fact mean life or death for some species as habitat quality can now reverse or just simply fail. The basis for much of our habitat quality in shallow water is largely determined by bacteria. It is the same shallow waters in which we harvest shellfish. Large shellfish populations in shallow water clear turbidity and allows ultraviolet light to reach the bottom, killing bacteria. That is why shellfish beds in cold clear water have lower bacteria counts; ultraviolet light kills bacteria. It is this natural condition that has developed UV light systems in aquaculture closed systems and the foundation of pool ultraviolet sterilizer filter units. I recall in August 2008 a trip with my son Willard from the Branford River to the Farm River. We took what is called the “Farm River Gut,” we left the warm brown turbid water of the Branford River and proceeded to the gut – the water was approximately eight feet deep, but it was Will who noticed the difference then beneath our Brockway skiff – at the time, a large oyster bed was below and the water was crystal clear and therefore ultraviolet light could penetrate the water column. Shellfish are tremendous filter feeders and clear, cool water allows light to kill bacteria. Heat and turbid water cause bacteria to thrive, and the changes in bacteria can be attributed to the penetration depth of ultraviolet light. More recently, aquaculture is not only considered for employment and food, but in addition, the ecosystem services oysters and clams may provide to shallow water.
When we look at blue crabs, we had a southern New England peak in 1912 and 2010, nearly a century apart and a small overlap in lobster peaks, 1878 and 1998. Both lobster peaks soon witnessed collapses in the south, and following the lobster collapses, blue crab surged in southern New England. Just a few degrees over a long period of time can have huge habitat differences for fish and shellfish. One of the climate signals is the return of the sulfur cycle, noticeably absent from many estuarine studies. And heat returns the sulfur cycle bacteria while cold increases the nitrogen/oxygen cycle bacteria. While we have concentrated concern on the release of sulfur from fuels (mostly coal), we have missed the greatest sulfur source in the sea – sulfate. Complicating this issue is that sulfate is also a potential source of oxygen, so also is nitrogen in the form of nitrite and nitrate. When elemental oxygen is limited, organisms, including bacteria, utilize them instead. For sulfate-reducing bacteria, they will never run out of oxygen complexed with sulfur, even with no elemental oxygen in seawater.
The emphasis in the past few decades is on the amount of elemental oxygen in seawater, which is very temperature dependent. Hot seawater naturally contains less “oxygen” than cold seawater. In hot seawater, bacteria that need oxygen look for other sources, such as nitrite or nitrate, and when that is expended, they die (waste water treatment plant operators know this as also those constructing biofilters for closed aquaria systems). A new bacteria then takes over but this bacteria is, in fact, very old - the bacteria that ruled before the earth cooled, the bacterial sulfate reducers of Vibrio. We can see the result of this on land, oxygen bacteria make it possible for plants to gain nutrient ions, in fact to grow, they sustained plant roots. Take away the oxygen bacteria and plants die, floods do that, so does heat but sulfur bacteria thrive. In terrestrial terms, plants have root rot (or failure), a sulfide black layer that forms without oxygen. The sulfur bacteria produce a plant toxin, hydrogen sulfide; it kills the plant by killing its roots. Salt marshes also exhibit this sulfide-sulfur cycle – sulfide failure, we call it “marsh diebacks” or “sulfide browning.” Both have a direct sulfur bacteria temperature cycle link. Some plants have the ability to leak oxygen to their roots, forming a safety shield, so to speak to keep its oxygen bacteria alive surrounding its roots. Some of our salt marsh plants can do this, so can eelgrass. This is because they live in a rich organic marine soil that at times contain both these oxygen and sulfur bacteria and both have adapted to bacterial biochemical responses that enable them to live in sulfide environments for a while, but not forever. That is why the discovery of these ancient sulfur bacteria, living in extremely hot ocean vents (black smokers), was so sensational in the 1980’s. It was so opposite our oxygen world, which needs cooler temperatures. In heat, the oxygen world (of which we share of course) declines as sulfur “wins.” These vibrio bacteria have been recently named – Vibrio antiquities, the vibrio of ancient times. Removing nitrate and nitrite in low oxygen conditions, in fact, removes potential “oxygen sources” from oxygen bacteria that need it, as sulfate-reducing bacteria displace those that need oxygen or nitrogen-oxygen compounds. A ratio of nitrate to ammonia shows this bacterial filter change in high heat.
One of the problems of sulfate reducers is that they are incredibly slow; they consume the plant tissue (organic matter) much slower than oxygen bacteria in terrestrial soils. For example, a fall leaf is usually broken down to organic flour by spring. In the marine environment, it’s several years. The production of sulfides, an extremely toxic substance, slows the process even more, and why trees under water do not rot – and in black sapropel, sulfide environments are preserved. Reclaimed trees lost in huge log drives in rivers two centuries ago still have bark attached and clearly visible axe marks. A similar tree exposed to oxygen is consumed by bacteria and shown by wooden boat builders as centuries of trying to stop cellulose-consuming bacteria, we call wood rot. When it gets hot, shallow waters experience this sulfide-sulfur bacterial war (See Environment & Conservation #7: Salt Marshes - A Climate Change Bacterial Battlefield, posted on the Blue Crab ForumTM, September 10, 2015). They consume organic matter (mostly leaves in our area) without oxygen, taking sulfate from seawater and freeing its oxygen from sulfur; as waters become acid, hydrogen combines with sulfur to form hydrogen sulfide gas (the rotten egg smells of hot summer nights). Coastal residents often refer to this as “marsh gas” as it is also emitted from the same bacterial process in salt marsh peat.
Some of the sulfide combines with iron, turns black and becomes the ferric sulfide sapropel or “black mayonnaise.” It is this process that now purges ammonia as bacterial decay continues to favor those bacteria that produce methane. These are sometimes termed the “primitive bacteria,” the methanogens, don’t need oxygen compounds at all; they live by stripping all molecules from the carbon chains of proteins, and in this process, release the most damaging “greenhouse gas” - methane.
In times of cold seawater, dissolved oxygen keeps these sulfur/sulfate bacteria populations low. In fact, oxygen and some of biochemical discharges can kill sulfur bacteria as some of the first antibiotics came from studying this bacterial war such as Streptomycin (Selman Waksman, Rutgers University).
Early Trees and Sulfur Mists
The first trees did not develop at a time that oxygen bacteria could reduce (consume) organic matter. It is suggested that in this transition the first coal was formed, rapid (hot) organic matter could accumulate and sulfur reducing bacteria could not consume it fast enough, and as layers of sapropel formed, these black deposits putrefied and underwent sulfate bacterial digestion. This is how sulfur got into coal, and why, when coal is exposed to air, sulfuric acid is formed and these long dormant bacteria start to eat again. In coal mines, when mining started and water cooled equipment leaked, some coal mines started to produce methane, the air and water “woke up these bacteria” that now started to “eat” the coal again. It is the Sapropel that builds in coastal areas is the first step in coal formation, and we can measure this by bacterial growths and the sulfides-sulfuric acid it produces when re-exposed to air - oxygen.
It is a feature of southern New England and Canadian Maritime coastal farm life and agriculture along its coast a century ago, the use of marine muds as soil nourishment-fertilizer. Fresh or surface deposits often called “mussel mud” were used on land to build up oxygen bacteria in the soil. Such organics or marine manures feed the oxygen bacteria on hay fields and salt marshes. That could be termed a marine humus, but deepened deposits sealed from oxygen was the realm of the sulfur bacteria, and these often had a blue-black jelly-like consistency. That blue-black description and rotten egg odors frequently appear in the fisheries literature as well as in agricultural reports. Farmers, who harvested cold water humus, reported increased hay field yields, but those with a blue-black jelly consistency formed sulfuric acid in air – and the low pH ruined crops and fields. Over time however, they slowly recovered and New England Experiment Stations urged farmers to add shell (oyster or lobster) to offset this “hurtful” acidity.
Following are remarks made by Mr. Stevens, a farmer in Essex, CT in the late 1870’s that have been quoted in a CT Board of Agriculture Report printed in 1879:
“Our mill ponds a few miles back from the river (Connecticut River – T. Visel) contain a rich, black mud, quite deep and with a very strong smell. It has been tried on various crops but kills everything. After being hauled and dried, it turns from black to white and puckers the mouth like alum!!”
New England Experiment Stations quickly tested these sapropels and found very high sulfuric acid levels and urged farmers to cut in lime or oyster shell to offset this “hurtful acidity” (See Connecticut Rivers Lead Sapropel Production 1850-1885, Blue Crab ForumTM, Fishing, Eeling and Oystering thread, September 29, 2014).
But what farmers and fishers experience is the battle of temperature fought by competing bacterial strains, the worst of all the Desulfovibrio species that have brought so much hardship to blue crabbers to our south. As waters heat up, these “vibrio” bacteria need the surface humus now turns to sapropel and sulfides build up – if released by storms and oxygen – you get the hurtful acidity of sapropel sulfuric acid washes (If you want to see this chemical reaction, view a short clip titled “Violent Soil” by soil scientist, Dr. Del Fanning, University of Maryland, June 27, 2013).
As climates change, cooler water with more oxygen penetrates sapropel, creating acids – that is what is suspected of creating the die off of eelgrass in the 1920’s (See Niantic River Studies IMEP #7: Niantic Bay Scallops Seed Transplants 1916 to 1935, A Question of Habitat Quality for Bay Scallops – An Account of the Niantic Bay Fishery, The Blue Crab ForumTM Fishing, Eeling & Oystering thread, February, 25, 2014 and IMEP #60: Temperature, Energy and the Niantic Connecticut Bay Scallop Fishery, Habitat Information for Fishers and Fishery Area Managers – Understanding Science Through History, The Blue Crab ForumTM Fishing, Eeling & Oystering thread, February, 7, 2017). As waters cooled, oxygen levels increased in thick sapropel sulfuric acids kills eelgrass roots, weakened as the plant tried to move more oxygen to its roots to keep its needed oxygen bacteria alive – further weakened it to a point that it succumbed to molds and fungus. After 1920, New England’s climate cooled from the extreme heat and cholera outbreaks (cholera’s full name is Vibrio cholerae – notice that the vibrio has been largely dropped from everyday usage) of the Great Heat of 1880 to 1920, it became colder and winters more extreme. Storms became stronger and more prevalent. The rise of bay scallops after 1920 would also signal the end of eelgrass habitat expansion and took shellfish managers by surprise that cold and storms would bring a valuable scallop crop back to Rhode Island shellfishers after many years of low or no catches at all. By 1924, Rhode Island Commissioners of shell fisheries – Chapter 209 “Of Scallop Fisheries Rhode Island reports (See IMEP #52: The Narragansett Bay Deep Water Bay Scallop Habitats 1870s, The Blue Crab ForumTM, July 27, 2015) - The colds and storms improved bay scallop habitats:
“To the Honorable General Assembly of the State of Rhode Island and Providence Plantations:
(Chapter 209) “Of Scallop Fisheries.”
“We have probably had the largest crop of scallops during the Fall season of 1923 ever known in the history of Rhode Island, at least not within the memory of the oldest inhabitant has such a fine crop, both in size and number, been harvested as has been taken since the first day of September, and this in spite of the fact that the Winter of 1922 and 1923 was one of the most severe that we have had in a number of years.”
Rhode Island fishery managers were surprised because the return of cold was precisely what bay scallops needed, and with the cold came increasing storm intensity. So much so that this also appears in the Rhode Island Records – large amounts of bay scallop seed now washed upon the shore or beach to be frozen and lost to the fishery. Programs were quickly established to move bay scallop seed to deeper waters. Lost to the record, of course, was that twenty years prior there were no scallops and this scallop seed transport need was not necessary (See IMEP #52: The Narragansett Bay Deep Water Bay Scallop Habitats 1870s, The Blue Crab ForumTM, July 27, 2015). The 1924 Report of the Rhode Island Shellfish Commissioners continues:
“We attribute it somewhat to the fact that during the late Fall of 1922 permission was given the Free Fishermen to move under the supervision of our Deputies seed scallops from the shallow water in shore, where they invariably are winter killed, to deeper water off shore, where the chances are good that they will survive the cold weather and become strong and healthy. As we stated in the 1922 Report, about 3,000 bushels were thus moved. From results obtained we feel that the move was a good one, and the experiment, if it may be called such, has been repeated this Fall, and at this writing something over 67,000 bushels of seed have been placed in deep water, taken from the shores, where it would inevitably die when cold weather came- more than double the quantity that was moved one year ago with such splendid results. In this work, we have received the hearty and earnest co-operation of the Association of the Free Fishermen, who have been indefatigable in their efforts to assist the Commission in conserving the supply of seed scallops as much as possible.”
Did fishery managers suspect that as it turned colder with more storms casting seed scallops that a bacterial battle was occurring at the same time in shallow, composted marine soils? I don’t think they did. However, as oxygen penetrated organic composts that built up during the heat, sulfuric acids now formed and caused the eelgrass that lived in it to “waste away” in a compost that was also transitioning. More energy and colder water would now favor the oxygen bacteria, not the ones that could live in sulfate proceeded by a sulfuric acid wash. Eelgrass now dies out or wasted away, leaving a blue-black foul smelling deposit frequently mentioned as “dead bottoms.” As storms increased (1938 Hurricane was a climate cycle wakeup call), sapropel was washed away and then the gray sands below it cultivated. With cultivation came decreases in fines (rock flour), clay particles moved into suspension and then out or away, and exposed the long dead remains of bivalve shell. Now water and wave hydraulics brought to the surface this shell as it is lighter than the sand, a natural pH buffering effect, but instead of separation gold from soil, it was now the shell. Soils that had obtained clays from erosion or leaves from rain/melt waters were suddenly and dramatically cultivated, rinsed, aerated and then limed (by bivalve shell hash) – perfect conditions for great Quahog sets – and the colder 1950’s and 1960’s are known for huge landings of the Quahog following a period of scarcity in Rhode Island for the oxygen bacteria they quickly now reestablished themselves. They had now “won” in the marine soils of colder temperatures and more oxygen as sulfate bacteria died off. This, in turn, opened the oxygen/nitrate pathway, producing large amounts of nitrate, which fed spring blooms of algae now needed to feed the quahogs.
We really don’t have what soil scientists measure for terrestrial soils, a good before and after look at marine soil cultivation. Until very recently, the term sediment was used to describe them, and by doing so, glossed over the important role of marine bacteria. If someone says “soil” to you, a picture of a field or garden comes to mind, perhaps lime application to control pH or the addition of compost or fertilizer to assist plants. Now picture “sediment” and perhaps the sink trap or water purification system or solids that settled from a liquid in a filter comes to mind instead.
Shellfish researchers a century ago did call it soil and mentioned the role of bacteria in them. It is the bacteria in soil that has waged war since the earth cooled with those that thrive in heat (no oxygen) and those that prefer cold (oxygen). This bacterial battle is an old one and one that we can measure in the soil itself. We already do this in terrestrial soils, and the smell of sulfur with wet sticky black layers in soil immediately below an athletic field can send turf management into crisis action. In the marine field, such black layers or sulfur smells remain a mystery?
The Viking culture perhaps is the best example of bacterial battles between oxygen and sulfur that we can document, the ability of sulfur reducing bacteria to complex heavy metals we know today as “bog iron.” Here surrounded by organic matter submerged by water sulfur (no oxygen) bacteria thrived and gathered iron ions released by acids into a solid mass or nodule. Many feel that this example is a most appropriate illustration of metal chelation, the ability of sulfur bacteria to collect metals, often termed Sulfur Reducing Bacteria (SRB). Connecticut, for example and my hometown of Madison, has a history of “bloomeries,” early smelt furnaces that produced iron for hinges, nails and farm tools from “bog iron.” In fact, road names such as Bog Iron Road or Iron Works Road, provide clues to the early harvest of these metal nodules, and Madison’s history makes mention of these bacterial by products, even today from bogs.
The Madison Land Conservation Trust, Madison, CT provided an excellent description of the Ironwoods Preserve in the 1700’s a bog iron bloomery for the public, which includes:
“In the late 1700’s, Henry Hill, Joseph Pincheon, and Redad Stone operated a bloomer iron works on Joshua Blatchley’s farm” (Madison was part of the Colonial colony of Guilford between New Haven and Saybrook). “Bog iron ore is a deposit of ferric hydroxides or limonite in the bottom of ponds, swamps or bogs. These deposits result from the act of iron fixing bacteria that decay the swamp vegetation. Deposits range from the size of peas and cotton balls to solid layers, most often colored reddish, dark brown and contained from 20% to 50% useable iron, interestingly, the ore can regenerate in 5 to 25 years, depending on the amount of decaying vegetation, the amount of iron in the water and on the condition of bacterial activity.”
George R. Lange, III, in completion of his masters degree program at Wesleyan University also thanks the Madison Land Trust for help with his thesis: The Trails of Madison – A Historical and Descriptive Approach contains this statement:
“After being washed, bog iron ore went directly into the fire. At a temperature of 2600oF to 2700oF, silicon based impurities melted and formed a liquid slag, closely resembling melted iron. Small lumps of bog iron ore were reduced to a malleable iron.”
The ability of these sulfur bacteria to complex heavy metals has been known for a long time, thousands of years.
In soil science, the aspect of pore soil space, the ability to move water and ions (plant nutrients) is often described as capillary action, large grains had more space (capillary capacity) than “fines” or small particles. These soils (large grains) were often termed well-drained and could hold air (dry) with oxygen deeper than those soils poorly drained. A quick example is, of course, water poured into a cap (with holes) of sand versus a cup of clay. Clay has such small pores that it is frequently used to line the bottom of ponds to store or hold water. Soils high in clay can retain water (a good soil attribute) but could remain wet for long periods rotting roots. Soils over time could become compacted and pore capacity reduced, and the addition or accumulation of clay over time (floods) could reduce soil fertility. The amount of or type of clay has long been an indicator of soil quality. Soils high in clay tended to need cultivation to allow air to keep the healthy, plant required bacteria alive. Flooded soils (for long periods especially in heat) were long known to reduce or even eliminate oxygen-requiring bacteria. These soils needed to be dried, re-oxygenated, and time given for bacteria important to plants to recover.
Nitrogen is the largest component of the earth’s atmosphere at 78%. So it is thought that the first plants didn’t need large root systems that, with increased rain, plants were able to grow in warm climates, access nitrogen from the soil surface, not requiring deep tap roots of the plants that adapted later to the cold. Instead, these plants had roots that spread quicker on the surface, and we know these species today as the grasses. This is why eelgrass moves quickly into shallow, cultivated marine soils; it helps start habitat succession and living in soils that may become, in time, oxygen-deficient and why it then can move oxygen to its roots.
Oxygen now comprises approximately 21% of the earth’s atmosphere, second largest to nitrogen, but divides the realms of oxygen (CHON – carbon, hydrogen, oxygen and nitrogen) and sulfur (CHONS), and the sulfur realm came first, the extreme heat that was ruled by the first bacteria, not plants or those bacteria that needed oxygen.
Sulfur bacteria live without oxygen and usually little noticed, they thrive in water soaked or submerged soils (marine) away from the oxygen-requiring bacteria except after storms. Here we have a natural soil cultivation event and the sulfur bacteria moved to the surface where they die. As sulfur bacteria create hydrogen sulfides when this happens, we can get the formation of sulfuric acid, often reported in the agriculture literature as severe drop in soil pH.
This storm cultivation event injects seawater, usually alkaline pH, and with the seawater comes oxygen. The rock flour of erosion or the clays carried by streams are released and suspended. This is the brown to slightly gray water after a Northeaster, here in Long Island Sound that people see. I can recall these southerly storms growing up in Madison and trying to surf these large “fun” summer waves off Hammonassett Beach, but the water was always brown, a result of the re-suspension of fine particles. In a few days, waters however cleared. It is a natural process.
I did not make the connection of waves or “energy” to soil renewal, habitat succession, even increasing pore circulation, or reducing clays; that would come decades later. I had cultivated garden soil, added shell to sweeten the pH, and noticed that plants grew better if the soil had a sand ingredient (low CEC). I did not think of marine soils having much the same impacts – they did and do respond the same way.
We really don’t have much information about the historical (modern) development of marine soil cultivation harvest practices, but we do know that it first appeared on Martha’s Vineyard (Nantucket) during the cold 1950’s when soft shells (clams) retreated into more stable soils in salt ponds. The 1950’s and 1960’s are known for constant storms; marine soils were constantly cultivated and only these well protected salt ponds held the remaining pockets of soft shell clams, now a species of value. These sub-tidal populations raised a harvest difficulty - how to separate legal clams from sublegal ones – and how to perform this task in water.
The introduction of water jet mine technology would forever change the knowledge of marine soils. Now for the first time after modest energy, the impact of storm waves could be observed by increases of shellfish growth. According to some of the first hydraulic clammers, including Mr. Frank Dolan of Guilford, CT, for the shallow waters, the impact of energy – once only observed after storms or from dredging project the movement of fines called “silt.” It was possible to see this impact in small areas, once before just seen only after a strong storm. After cultivation from hydraulics, quahog sets increased and clams grew better and had thicker shells. In areas of shell hash, bits of old shell now lay on the surface similar to lime on terrestrial soils – clam sets then were often heavy. The water was cloudy and full of suspended “silt” for a few minutes, but the smallest of clay and minerals, rock flour, was carried off by tides and moving water. Powdered rock, the result of which measures geological time itself, the powder created by rock against rock movement during glaciers moved with tides fills soil pores. Over time, these “fines” and clays washed from land settled out in the estuaries. Tannin, a natural flocculent from wood tissue and leaves, is also part of the marine soil discussion process. Areas of slow or low flushing both leaves and fines settle out and waters cleared. Clear water now allows ultraviolet light to kill bacteria. Clear tropical waters are frequently those low in bacteria. Tropical waters and ultraviolet light therefore impact disease prevalency. Any filter mechanism, marsh plants or submerged vegetation act to clear waters by sticky bacterial films similar to filter pack media found in today’s aquaculture systems. This is a natural process that also has a role in vibrio bacteria growth in warm waters. Vibrio pathogens that kill coral can live in the filter organic matter below submerged aquatic grasses. It is a sulfide vibrio that is bleaching so many coral reefs in hot seawater with organic matter. It is termed Vibrio shiloi. Black band disease (Vibrio coralliilyticus) has also impacted coral reefs now linked to the family of sulfide bacteria.
As waters warmed in Long Island Sound, vibrio shellfish closures occurred here as vibrio in heat “leaked” into the water column and were ingested by shellfish. Researchers have found that blue crabs also contain vibrio and vibrio infections have killed several blue crabbers according to media reports. We may find that the blue crab could become an indicator organism for vibrio because it spends so much of its life cycle in shallow water. In states to our south, vibrio monitoring is now taking place and that the amount of vibrio is related to both heat and the presence of organic matter. Eelgrass especially is now being studied for its ability to foster vibrio growths. Submerged aquatic vegetation root fields (peat) hold reservoirs of vibrio bacteria and therefore subject to a potential monitoring program.
Some of the most deadly vibrio bacteria strains are being found under eelgrass and submerged aquatic vegetation (See Sediment and Vegetation as Reservoirs of Vibrio vulnificus in the Tampa Bay Estuary and Gulf of Mexico, Chase et.al., Applied and Environmental Microbiology, 2015). The Sound School has issued three warnings about vibrio in deep organic deposits – the Sapropels (referred to as “black mayonnaise by fishers) that last warning being dated February 1, 2016 posted on the Blue Crab ForumTM, NE Crabbing Resources thread, titled “Important Notice for Blue Crabbers – Estuarine Researchers.” Anyone working with sapropel, deep organic layers sealed from oxygen, runs the risk of being close or in contact with vibrio species.
But it is the shallow waters that may give us a good habitat quality indicator; they warm up and cool quicker than large bodies of water and we may be able to “watch” or monitor this rise and fall of vibrio bacteria. Some researchers on Cape Cod looked at this increase in vibrio as our climate started to moderate after 1972 (Positive NAO phase). It is the heat and presence of sapropel that appear to govern vibrio.
In addition, many environmental organizations across the country are looking at clear water and ultraviolet light penetration as nature’s natural bacteria control. The ability of shellfish aquaculture operations or natural shellfish beds to clear water (termed environmental ecosystem services) is now looked at as allowing light to penetrate shallow waters. This adds an important monitoring estuarine factor and vibrio bacteria may become a key monitoring factor for shallow bays and cover estuarine habitat health. Some studies have already reported that the increase in shellfish aquaculture naturally increases filter capacity, and in doing so, ultraviolet light penetration deadly to bacteria is enhanced (Most domestic filter residential pool systems contain a sealed ultraviolet light unit for this purpose). It is evident that the blue crab can live in this environment and with vibrio and we should look at how this happens.
The type of bacteria species in marine soils may also become an important indicator of temperature and energy shifts from changing climate patterns. This research may help communities reopen closed shellfish areas long closed from high bacteria counts. Enhancement of shellfish aquaculture and water clarity as it relates to bacteria growths is something to consider for estuarine monitoring studies in the near future – my view, Tim Visel.
I respond to all emails at tim.visel@new-haven.k12.ct.us. |
|
Back to top |
|
|
|
|
You cannot post new topics in this forum You cannot reply to topics in this forum You cannot edit your posts in this forum You cannot delete your posts in this forum You cannot vote in polls in this forum
|
|